The Old New Thing
Practical development throughout the evolution of Windows.
Latest posts
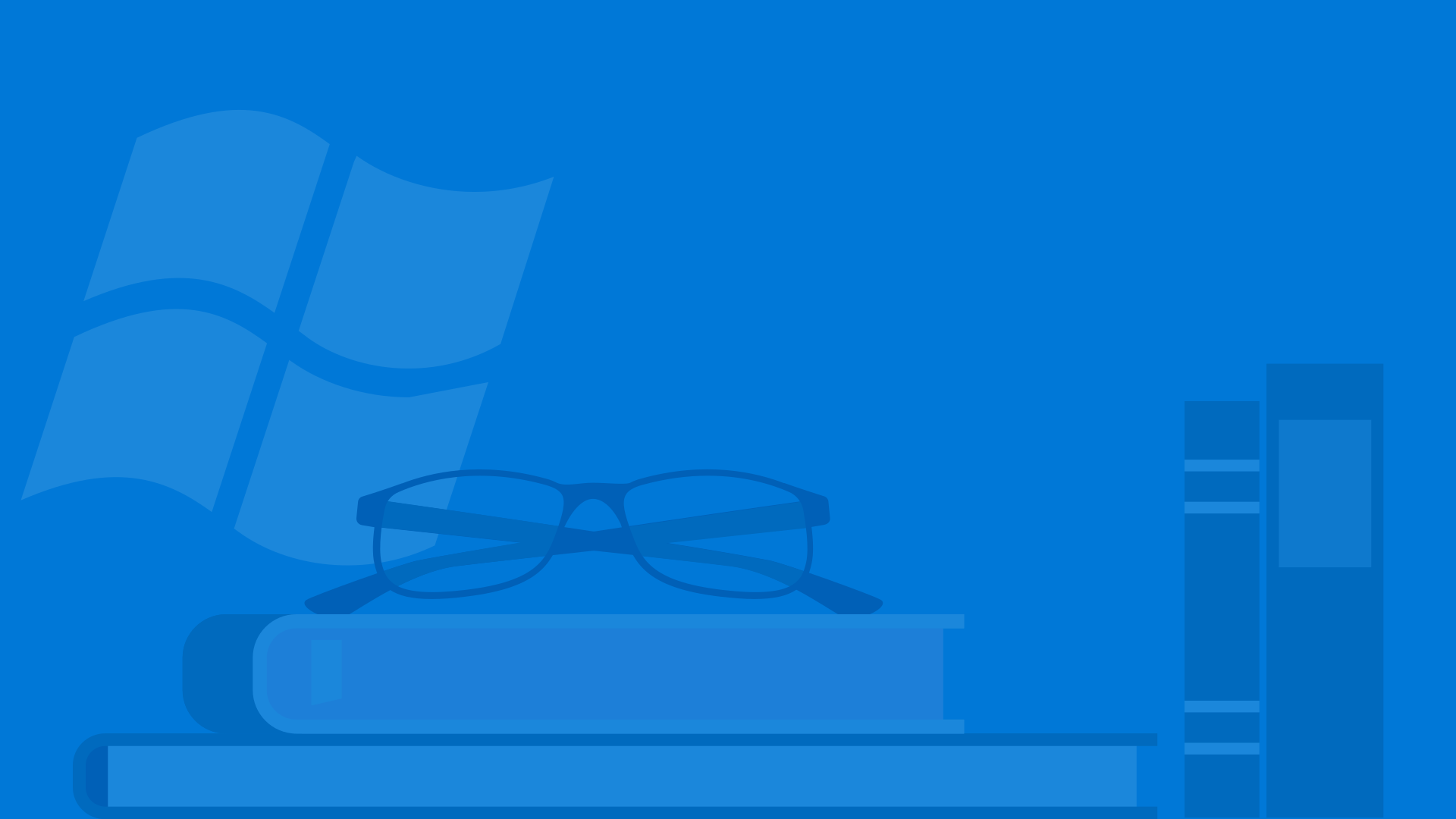
Jun 26, 2025
Post comments count
0
Post likes count
0
Why do I get errors about some weird symbol called ?main@@YAHP$01E$AAV?$Array@PE$AAVString@Platform…, part 2
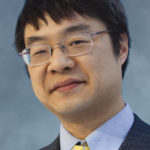
Figuring out why C++/CX makes a difference.
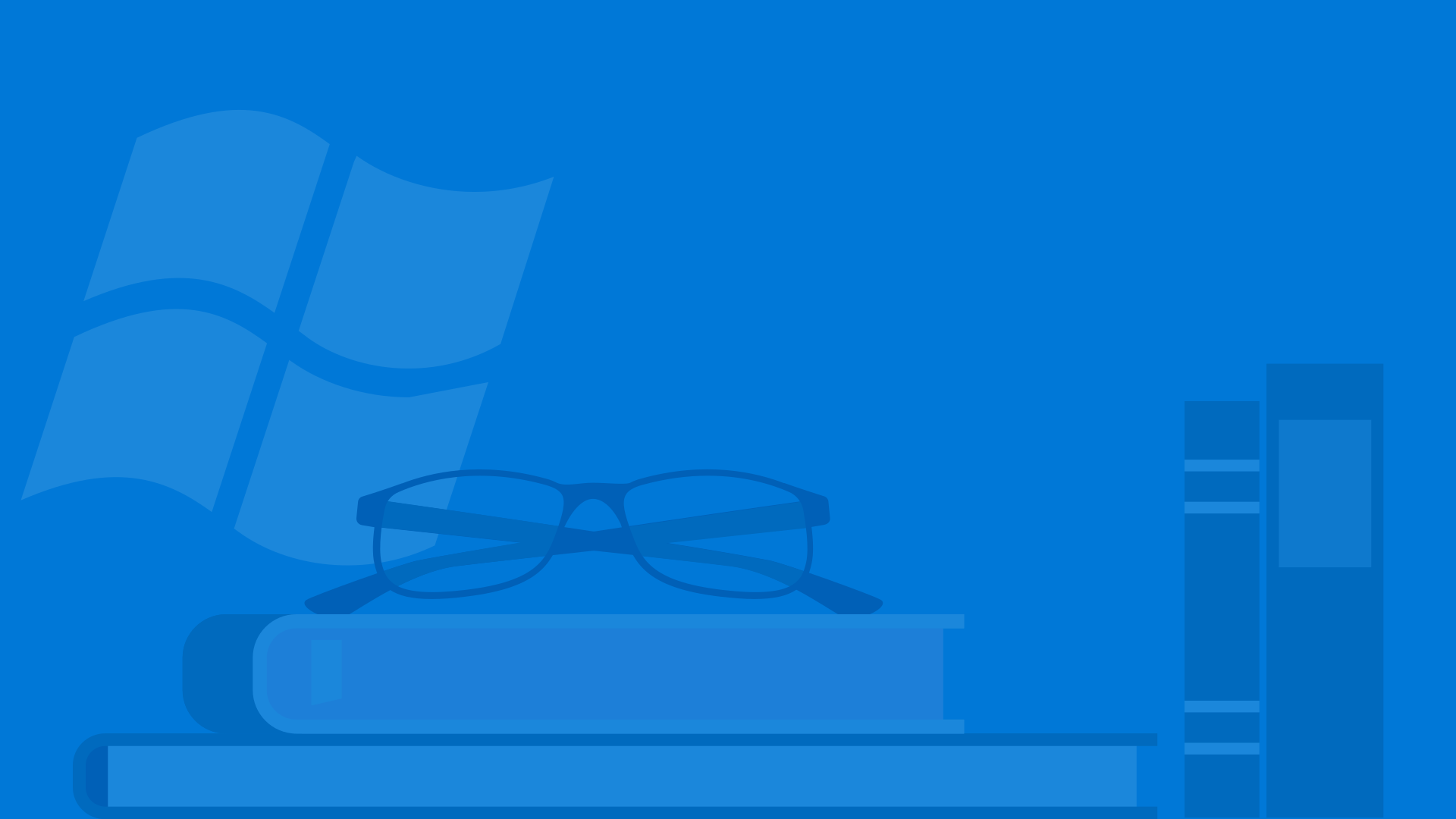
Jun 25, 2025
Post comments count
8
Post likes count
2
Your information has been permanently deleted, for small values of permanently
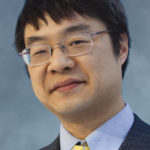
Is it really gone?
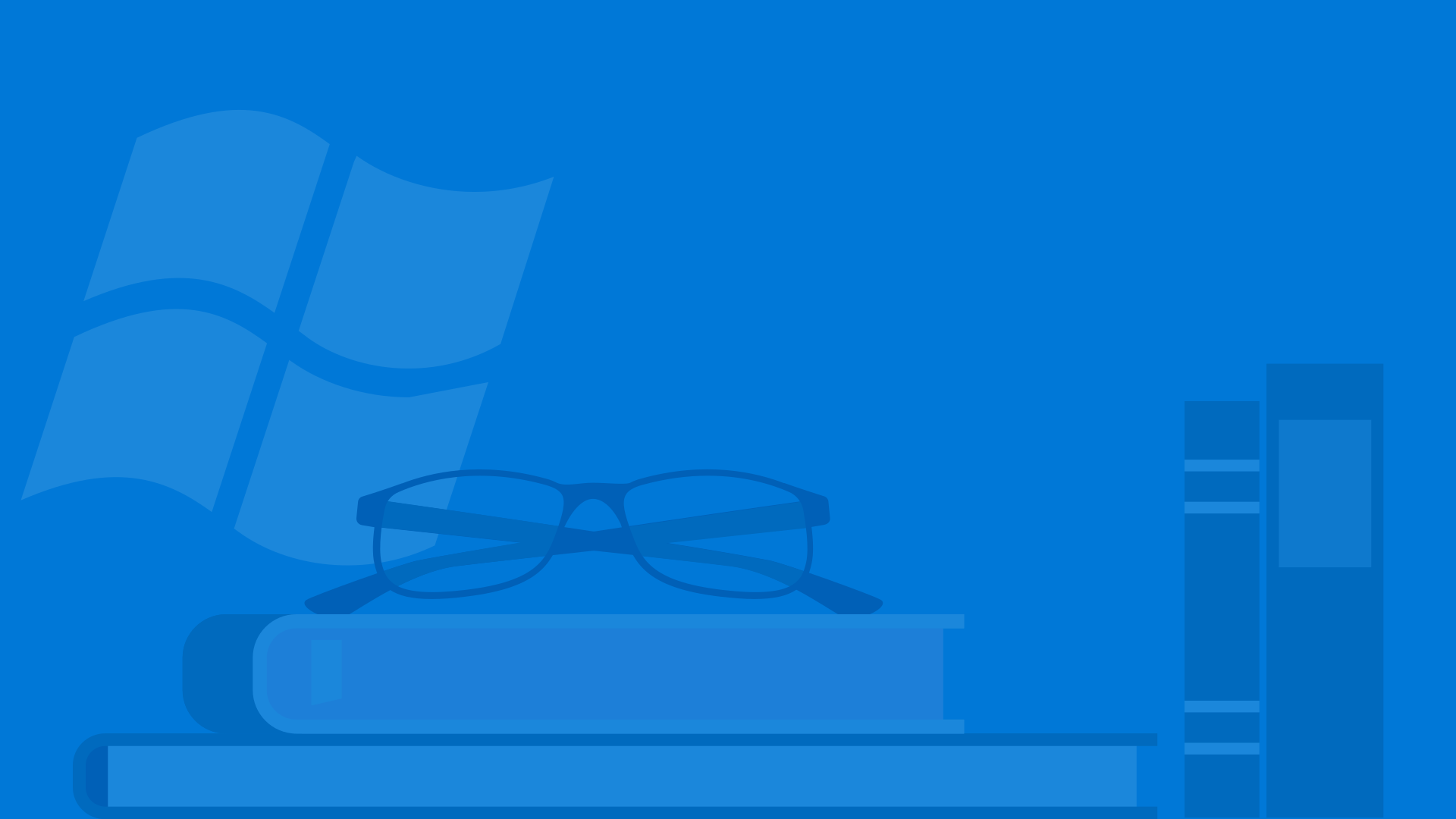
Jun 25, 2025
Post comments count
1
Post likes count
0
Why do I get errors about some weird symbol called ?main@@YAHP$01E$AAV?$Array@PE$AAVString@Platform…, part 1
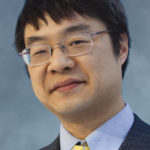
Welcome to the world of C++/CX. Is "welcome" the right word?
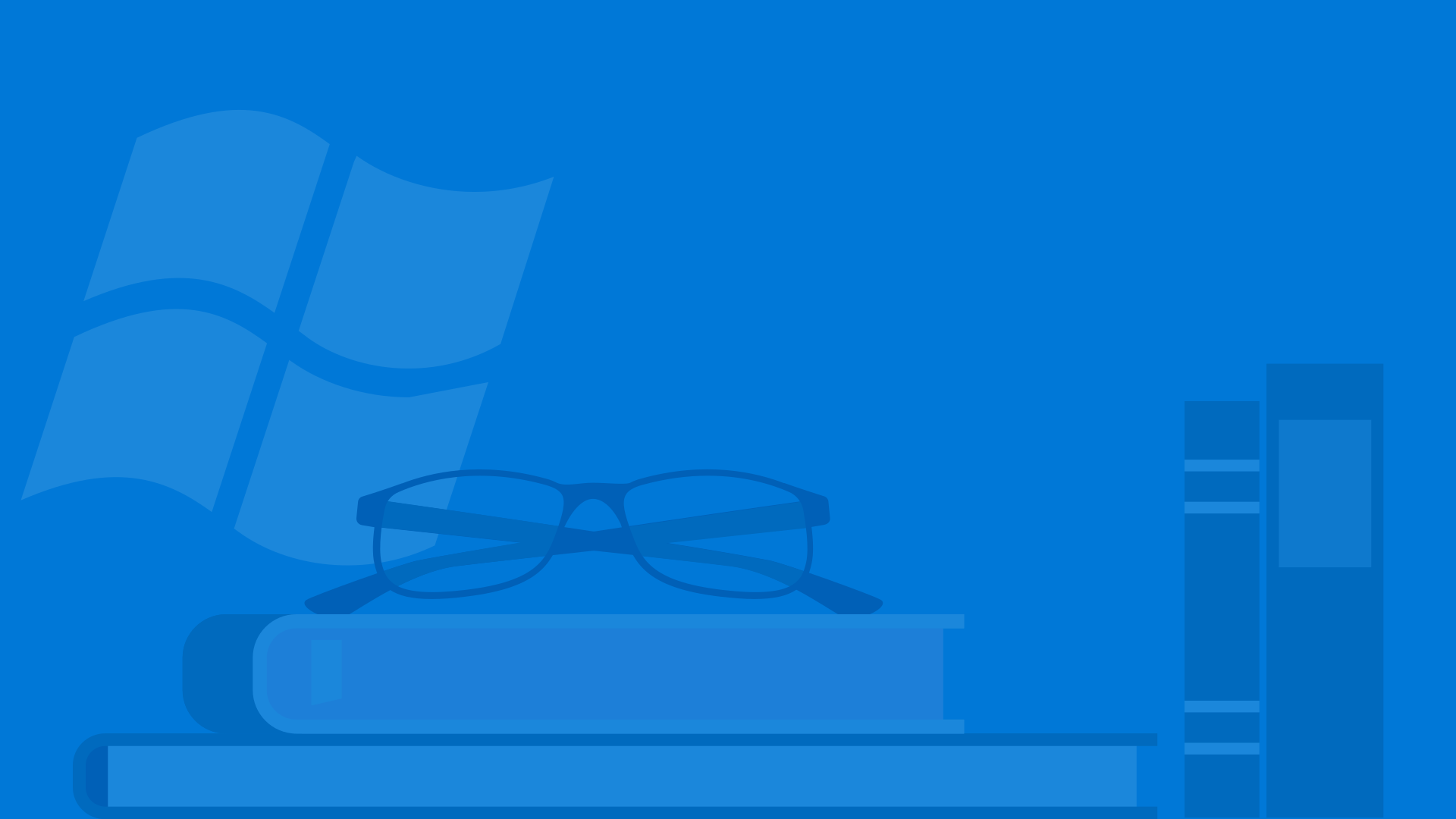
Jun 24, 2025
Post comments count
10
Post likes count
3
Abusing copyright strings to trick software into thinking it’s running on your competitor’s PC
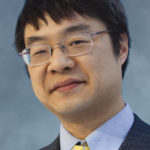
I did technically cross my fingers.
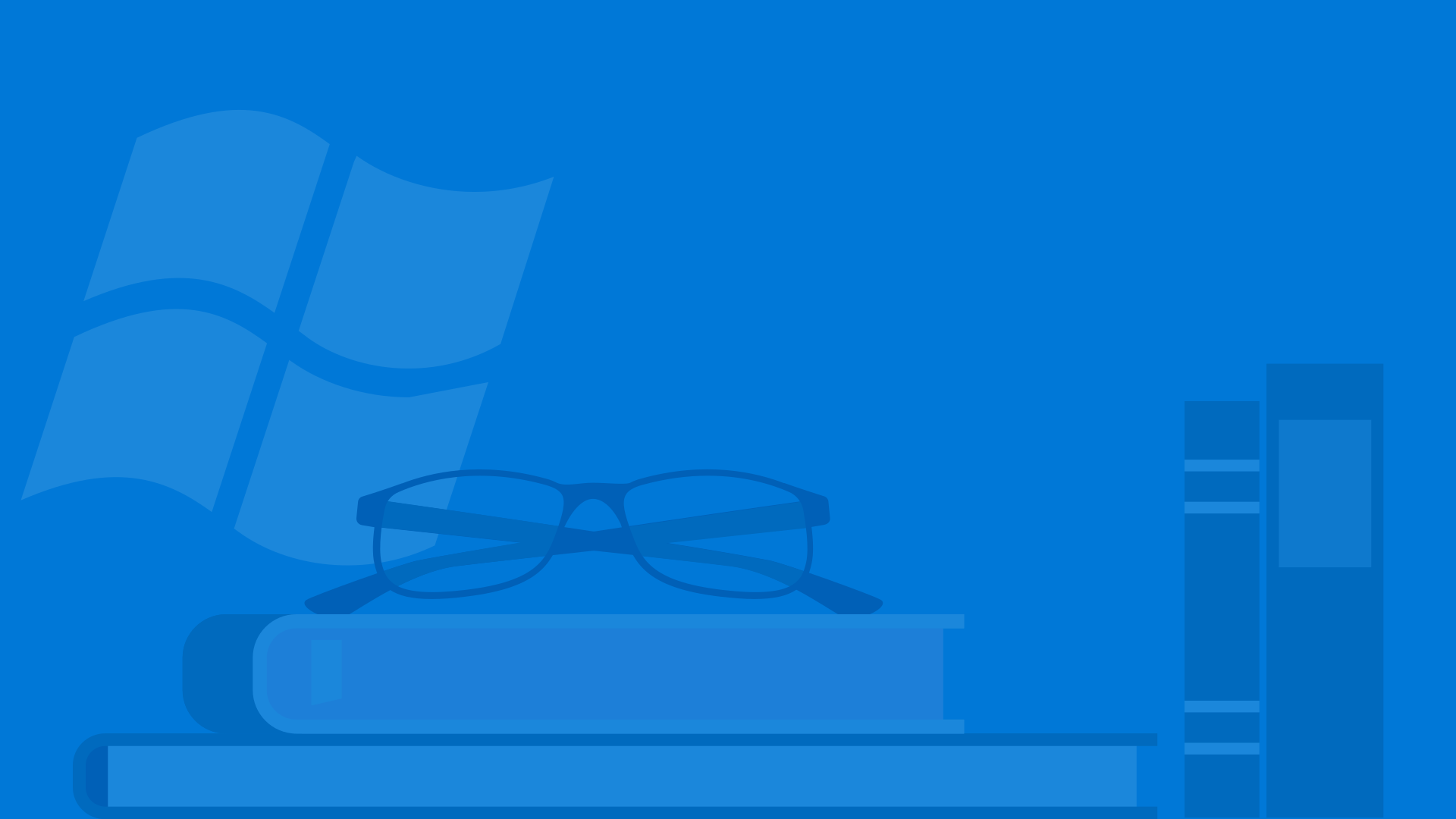
Jun 23, 2025
Post comments count
7
Post likes count
4
The MIDL compiler still has trouble with double greater-than signs, sadly
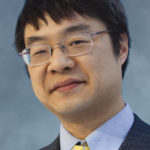
Many have tried.
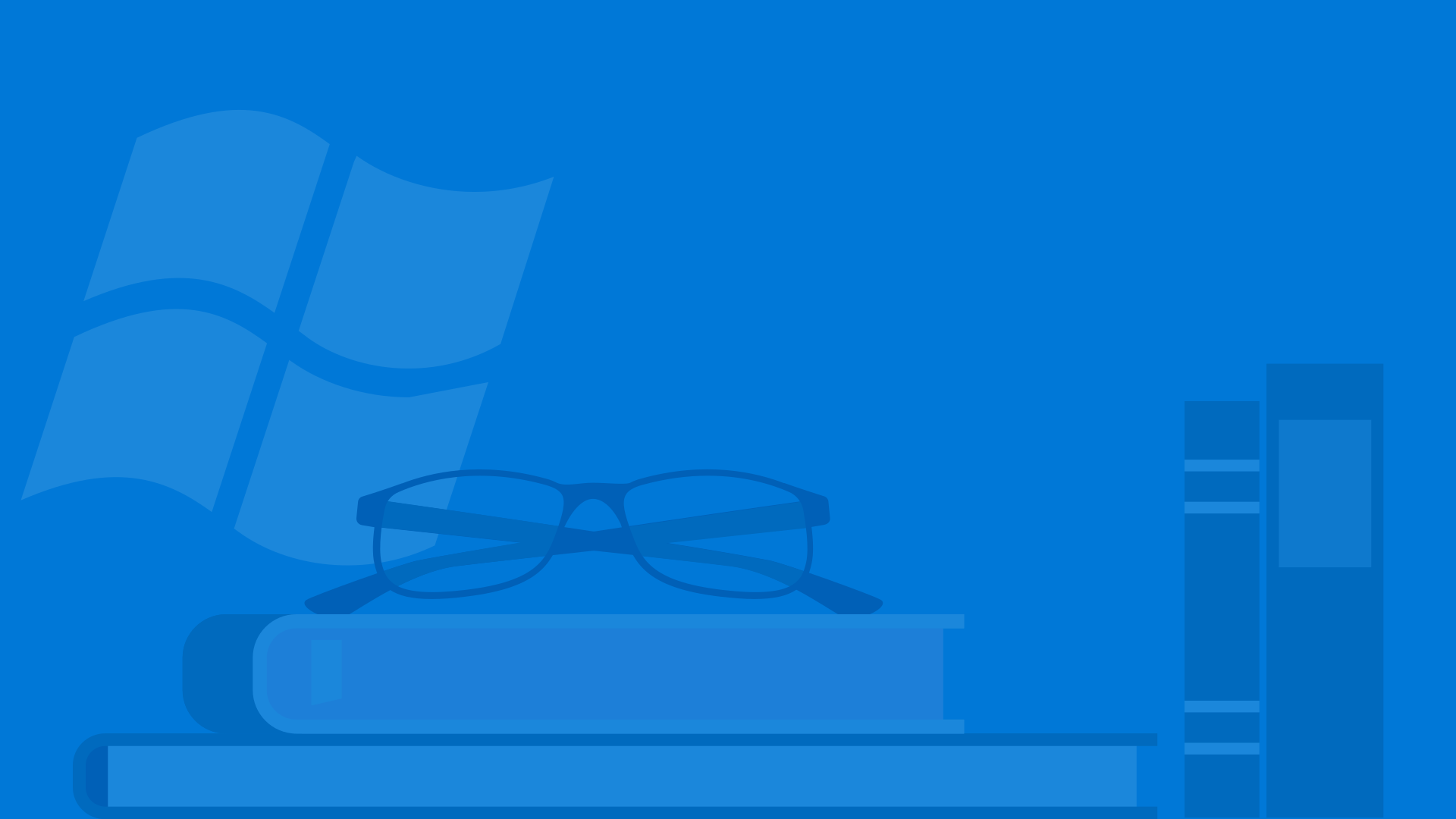
Jun 20, 2025
Post comments count
2
Post likes count
5
The case of the invalid handle error when a handle is closed while a thread is waiting on it
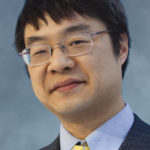
You are theorizing one race but experiencing another.
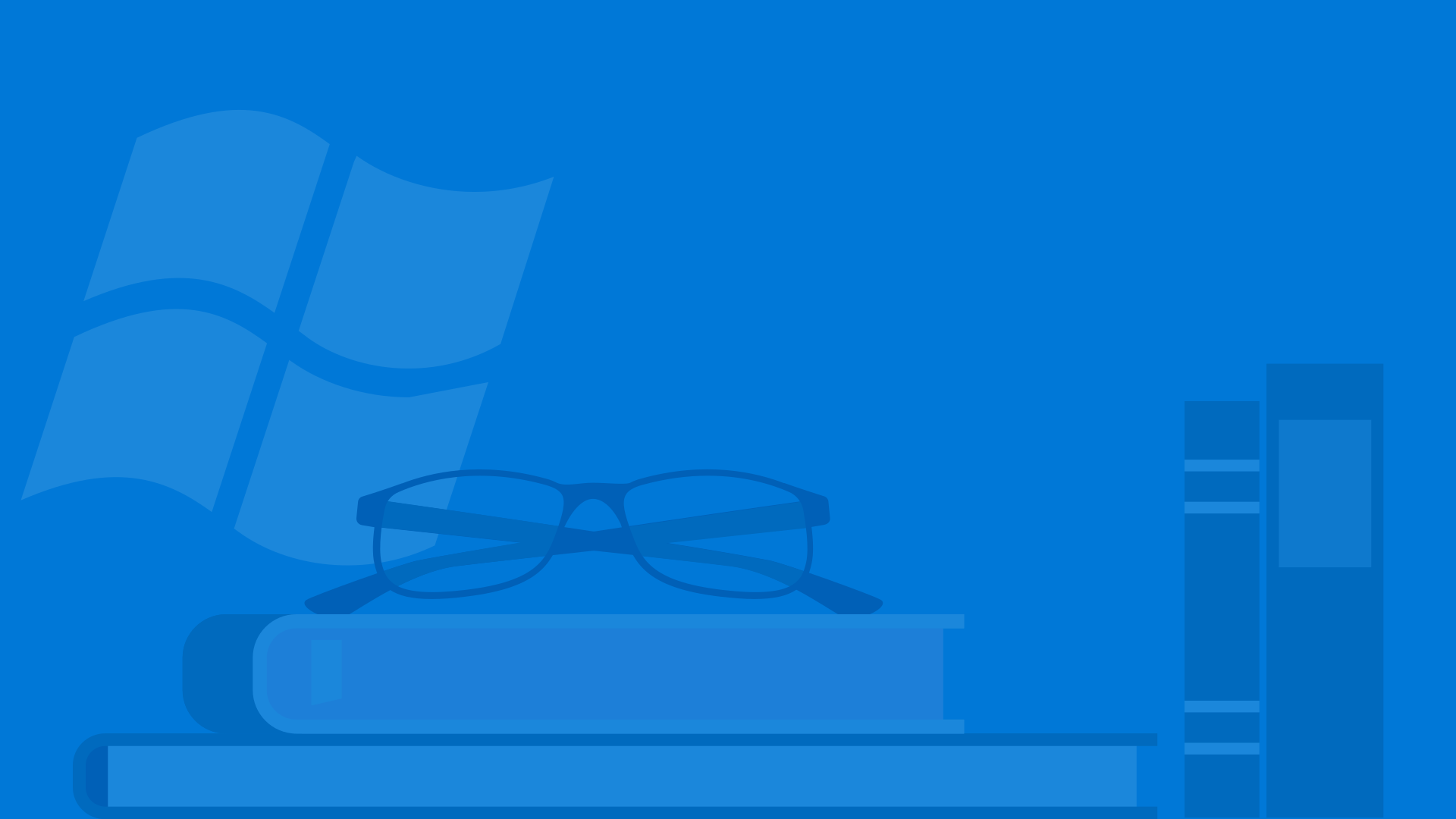
Jun 19, 2025
Post comments count
2
Post likes count
0
Learning to read C++ compiler errors: Ambiguous symbol errors after including a header file
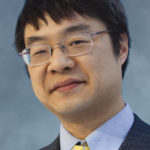
Finding out why multiple entities with the same name are visible.
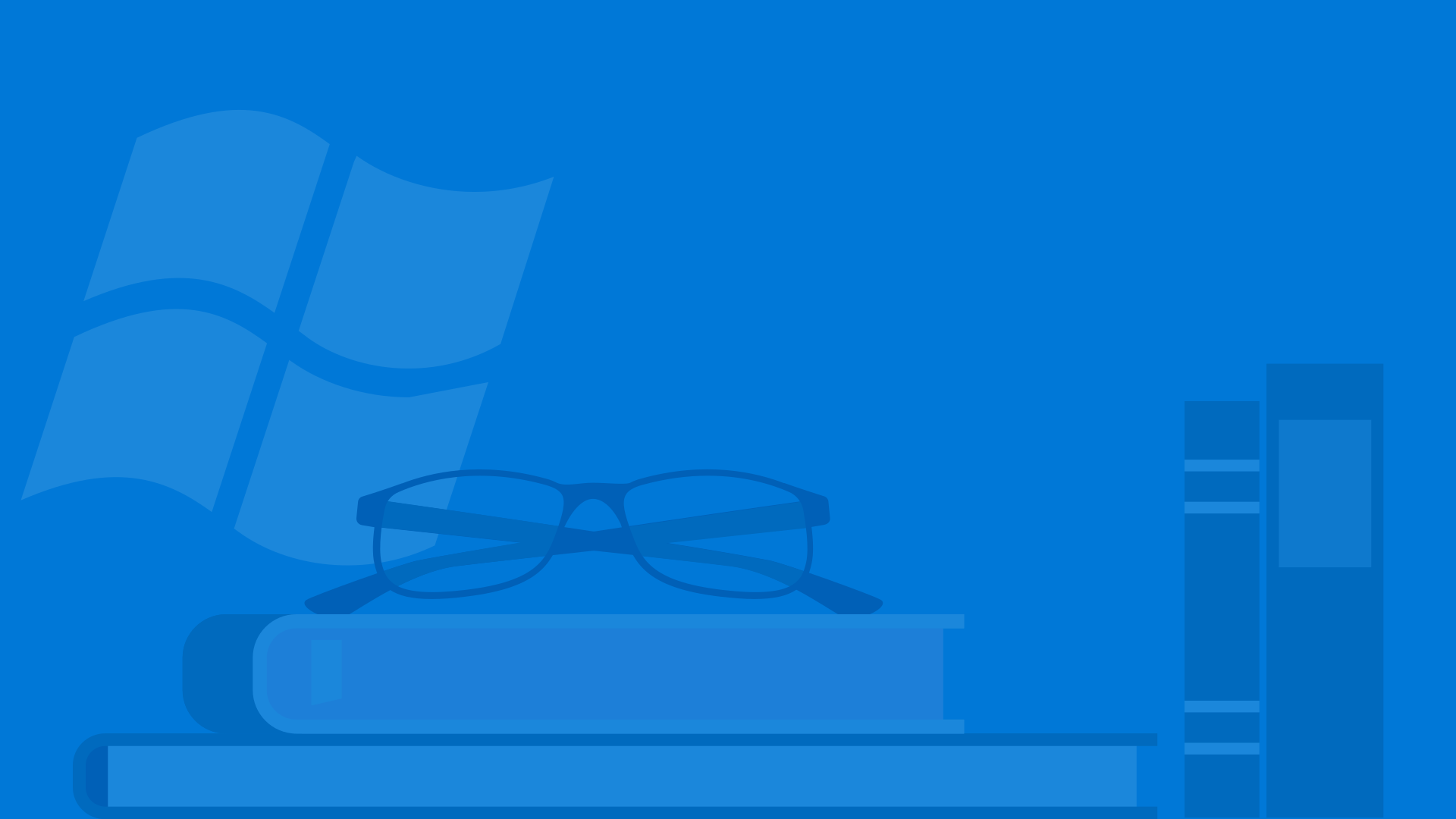
Jun 18, 2025
Post comments count
0
Post likes count
0
You have to tell Get-
and SetSecurityInfo
the object type, you can’t make it guess
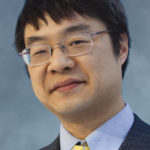
It needs to know which provider to give it to.
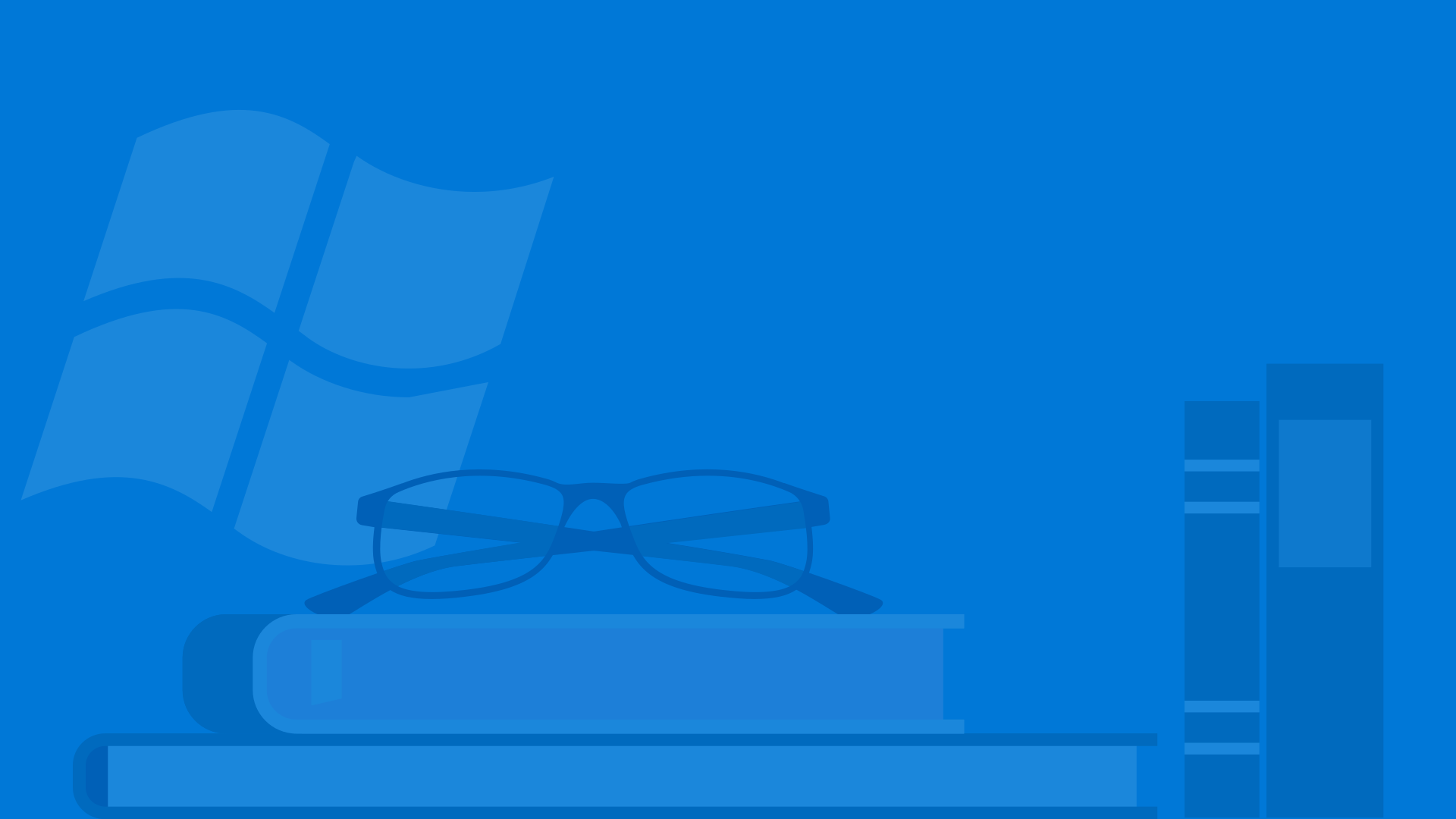
Jun 17, 2025
Post comments count
0
Post likes count
1
Funding the Egghead store shopping spree took a little extra legwork
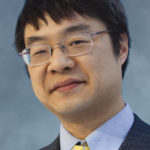
Just ask the boss.